In the late 19th century, neuroscientist Santiago Ramón y Cajal peered through a microscope at the spinal cords of three-day-old chick embryos and drew what looked like dust mites infiltrating a tomato slice.
Many at the time considered the brain a mass of continuous fibers, but Cajal believed the furred structures he was observing were discrete cells organized into relay teams with specific functions.
As he puzzled over how the cells formed connections up to a meter long, he came up with the notion of what would later be called growth cones, “a concentration of protoplasm of conical form, endowed with amoeboid movements,” he wrote.
Cajal hypothesized that neurons sprouted filaments with finger-like, motile protrusions at their tips. These could translate chemical cues into instructions that guided the axons to their targets. The idea unlocked an entire field in neurobiology that has captivated scientists for over a century.
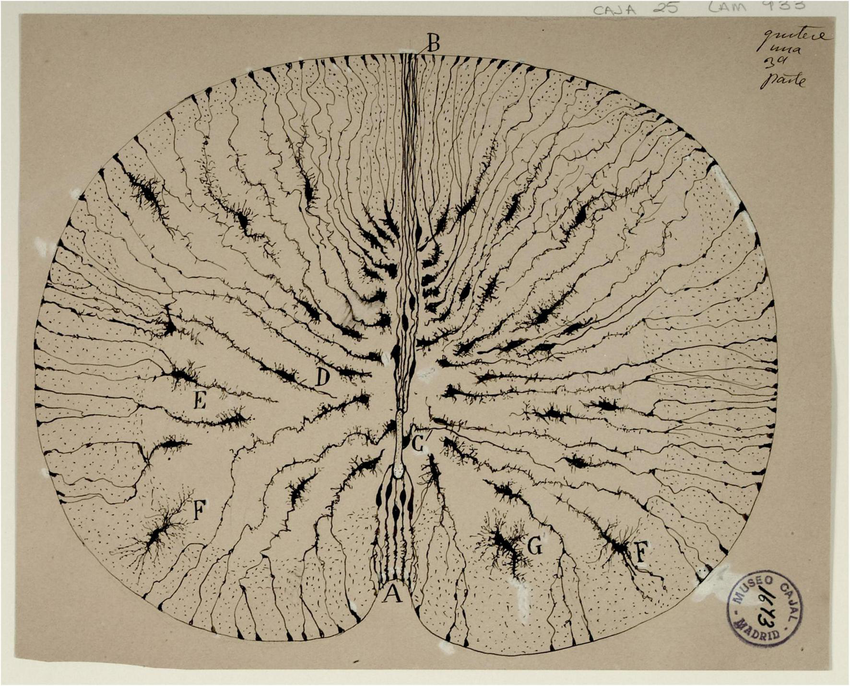
“It’s a beautiful picture, and why I started studying it,” says Rob Meijers, head of neuroscience at the Institute for Protein Innovation (IPI). “These neural axons are trying to find their path, and they’re helped along by these guidance cues.”
Unfortunately, it turns out that neuronal wiring is not that simple. There is deep complexity that needs far more work to understand.
Now, IPI aims to boost that research by developing antibodies targeting axonal guidance receptors and ligands.
The antibodies will enable the development of neuronal markers for all cell types, the tagging of neurons to track receptor activity in a migrating axon and the manipulation of specific receptor-ligand interactions, to name a few applications. Such research will help unravel the signals and processes of axon guidance, and that understanding could have profound implications in neurodegenerative diseases, post-injury brain repair, cancer and more.
Controversy and complexity
The axon guidance story began in the mid-20th century. Neuroscientists post-Cajal were divided into two camps: one claimed neuronal development was preordained and specific, while another postulated that axons grew randomly, connecting when they happened to “fit” the molecular specifications of a target cell.
Studies eventually turned up a more complicated interplay. At least four long- and short-range mechanisms — contact attraction, chemoattraction, contact repulsion and chemorepulsion — can trigger diverse and sometimes opposite responses. These position the axon with the challenge of navigating competing forces and signals at the same location during the same developmental period.
Similarly, researchers came to understand that there are also molecular signals, chemo-attractants and repellants that coordinate, integrate and direct axons along a safe path to construct a precisely wired nervous system.
The first neuroscientist to identify such a molecular signal was Marc Tessier-Lavigne, then at the University of California, San Francisco. He isolated in chick embryos an axon-luring substance he named netrin, which he and others later linked to an unlikely partner, a receptor called Deleted in Colorectal Cancer (DCC), first associated with tumor suppression.
Subsequent genetic analysis by Corey Goodman, a biotech entrepreneur and adjunct professor of neuroscience at the University of California, Berkeley, and others unleashed a succession of findings on guidance factor families and their receptors.
“Lots of receptors, lots of regulation”
To date, researchers have identified at least four canonical families of proteins involved in axonal pathfinding: netrins, semaphorins, ephrins and slits.
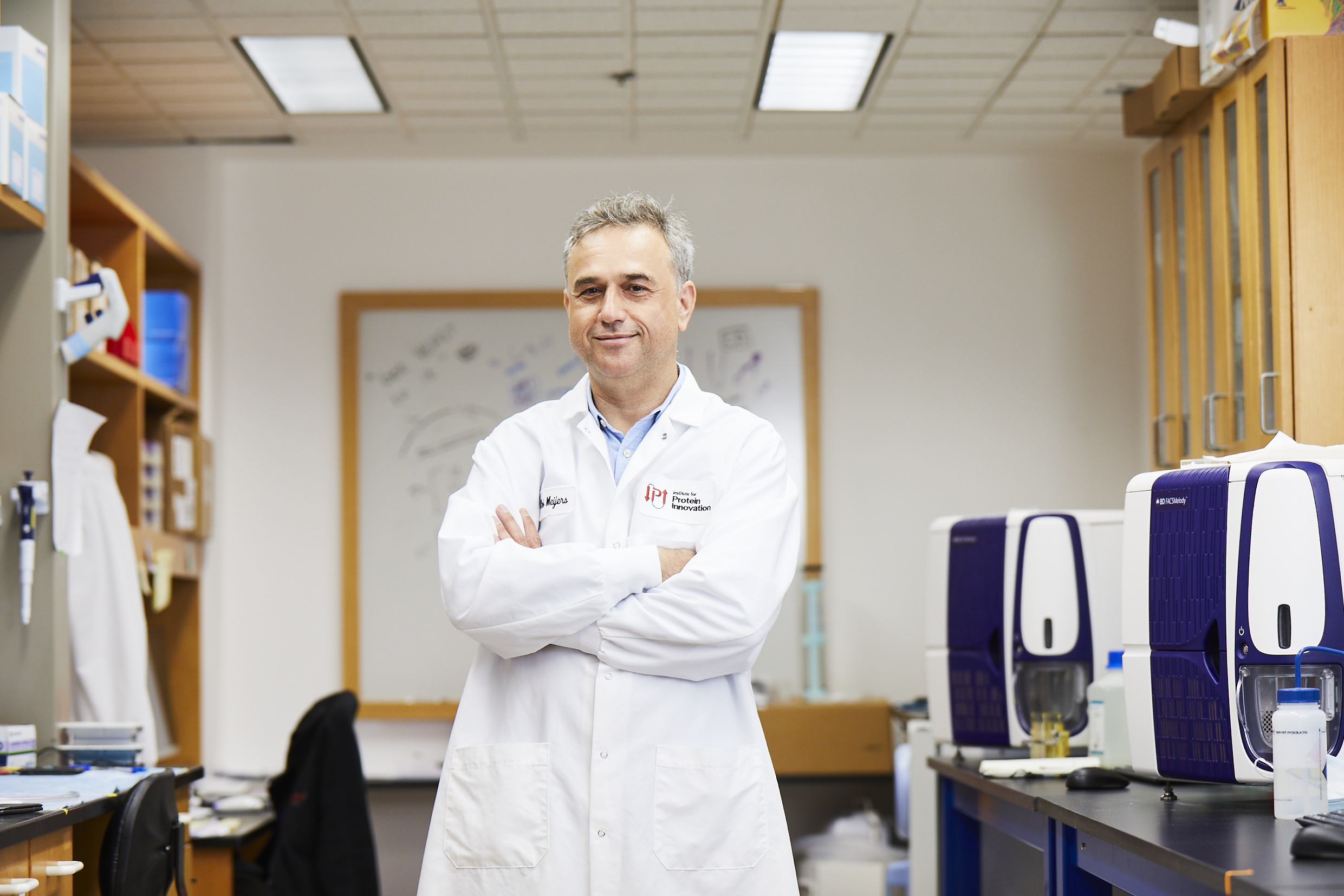
Netrins attract axons toward specific targets and repel them from others. In addition, they play a role in cell migration, morphogenesis and angiogenesis. Netrins interact with receptors such as DCC and UNC5, triggering signaling pathways that influence cytoskeletal dynamics and cell movement. For example, Netrin-1 guides commissural axons toward the ventral midline of the developing spinal cord. Axons need to be attracted to the midline in order to cross, but are then repelled from the midline to continue the journey to their target.
The large family of proteins dubbed semaphorins (referencing a signaling system used in rail and maritime transportation) were initially identified as repulsive cues. The more than 20 types of semaphorins identified to date play roles in cardiogenesis, angiogenesis, vasculogenesis, tumor metastasis, immune cell regulation, vascular development and other processes.
Semaphorins engage plexin receptors, which then activate downstream signaling pathways that affect cell motility and cytoskeletal organization. As cell surface receptors and ligands, semaphorins and plexins regulate cells in neurodevelopment, angiogenesis and immune responses. Specifically, Semaphorin 3A guides axons in the developing visual system.
Ephrins are cell surface ligands that bind to Eph receptors, the largest known family of receptor tyrosine kinases (RTKs). They play critical roles in developmental tissue patterning, neuronal connectivity, synaptic plasticity and cell-to-cell communication. Eph receptors and ephrin genes are associated with angiogenesis and metastasis in many types of human cancer.
Ephrin/Eph signaling can be bidirectional, influencing both the ephrin-expressing cell and the Eph receptor-expressing cell. This signaling can lead to changes in cell adhesion, migration and cytoskeletal organization.
Antibodies targeting Eph receptors, particularly EphA2, have been developed and are being tested in clinical trials to potentially trigger antibody-dependent cell-mediated cytotoxicity against tumor cells.
Slits are secreted proteins that act as repulsive cues, particularly at the midline of the developing nervous system. They play a role in axon guidance, cell migration and tissue barrier formation.
Slits signal through Robo receptors, which trigger downstream pathways that affect cell motility and cytoskeletal organization. Slit-Robo signaling helps prevent axons from crossing the midline multiple times, ensuring proper neuronal wiring.
A fifth duo, cell adhesion proteins teneurins and latrophilins, have more recently emerged as “new kids on the block.” They work together to regulate cellular communication and neural development.
Illuminating often overlooked work
As these and other molecular players debuted and fell under scrutiny, the mechanism of how axon guidance receptors guide axons began to evolve.
It began with the simple idea of a relay between receptors and ligands, with netrin as a far-off beacon guiding axons to grow toward it across hemispheres of the spinal cord or brain. The concept — and apparent technical feats to achieve it — became an awe-inspiring chapter in neuroscience courses around the world for decades.
But more recent genetic tools revealed that the axon guidance story has a different ending.
“It’s a heartbreaker,” Meijers says, “There are lots of people mourning that this fairytale is no longer true.”
A new story is emerging, with netrin demonstrating other modes of action, with different long- or short-range activities depending on the cell type. There are also more molecules showing up to play cameo roles.
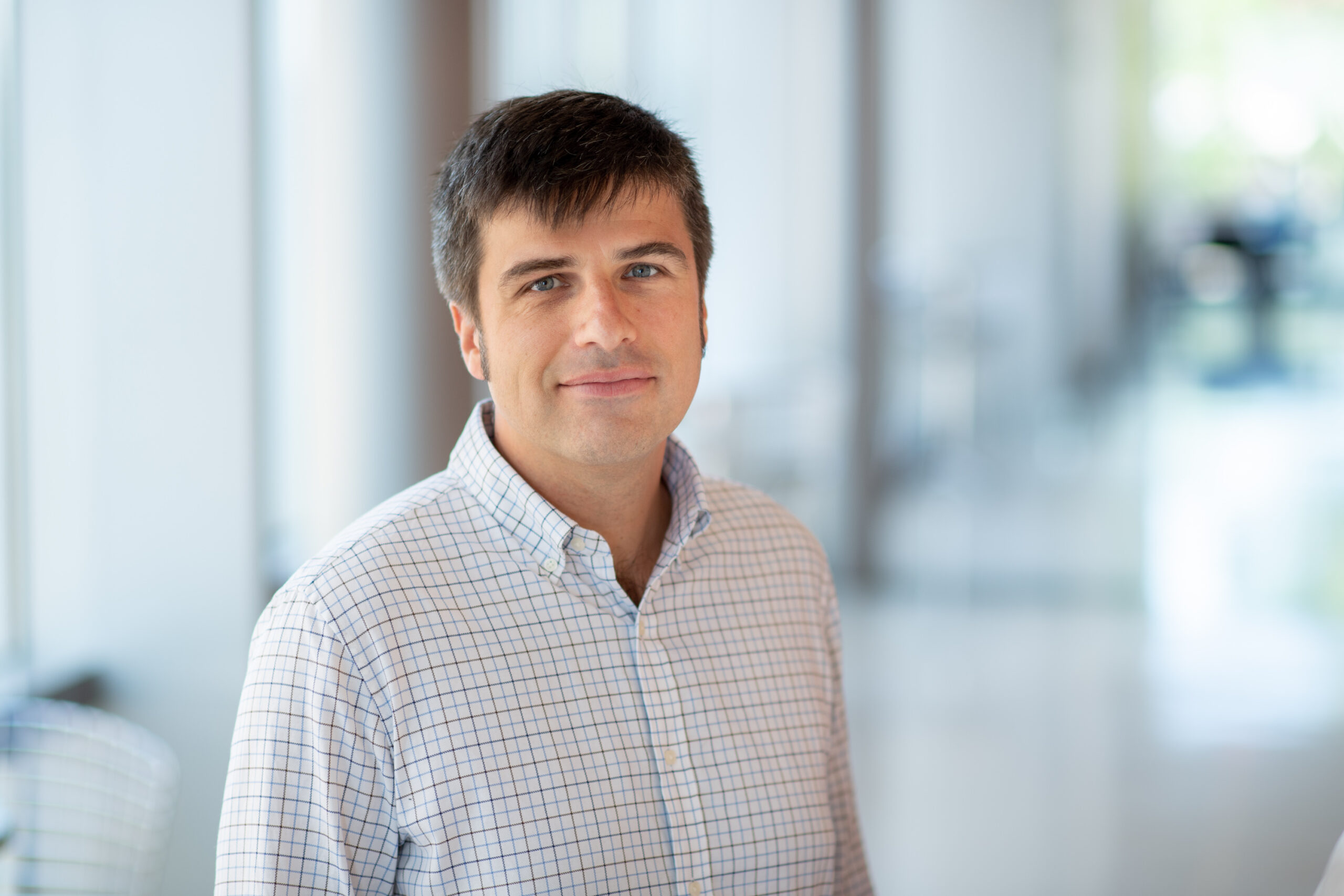
In a March 2025 Current Opinion in Neurobiology review, Meijers — with IPI principal scientist Shaotong Zhu and colleague Alexander Jaworski, an associate professor of neuroscience at Brown University — reported that certain ligand-receptor pairings defy the four-family dogma. Instead of a simple relay, it appears that many receptor-ligands work in concert, mixing and matching partnerships and bringing in even more of those molecular players to push and pull axons along.
In addition, increasing evidence points to the role environmental factors play in switching ligands and receptors on and off, a finding Cajal predicted long ago.
These latest revelations stem in part from large protein-protein interactome studies, done by “groups that are traditionally overlooked by the axon guidance community,” Meijers said. “We feel it’s important to make people aware that this data is available and reliable.”
Much more effort is needed to fully understand the complex, fundamental processes underlying the central and peripheral nervous systems and to translate that knowledge into future cures.
To that end, Goodman has noted — at the presentation of the 2020 Gruber Neuroscience Prize — that there are “lots of receptors, lots of regulation [and] lots of room for people to make further discoveries.”
To help researchers elucidate the roles of these proteins in the formation of the nervous system and pave the way for potential new treatments for neurological diseases and disorders, IPI plans to release netrin/DCC and Robo/Slit antibody panels in the coming months. IPI’s semaphorin/neuropilin/plexin antibody panels are also currently being validated through the Institute’s validation efforts. Additionally, a community-driven validation campaign for ephrins is planned for summer 2025.
Source:Rob Meijers, [email protected]
Writer: Deborah Halber, [email protected]
Feature Image Caption/Credit: Key known proteins, receptors and interactions involved in axon guidance. IPI figure by illustrator Anne-Lise Paris (www.in-graphidi.com).
About IPI
The Institute for Protein Innovation is pioneering a new approach to scientific discovery and collaboration. As a nonprofit research institute, we provide the biomedical research community with synthetic antibodies and deep protein expertise, empowering scientists to explore fundamental biological processes and pinpoint new targets for therapeutic development. Our mission is to advance protein science to accelerate research and improve human health. For more information, visit proteininnovation.org or follow us on social media, @ipiproteins.