Snynaptic function involves the interactions of hundreds, maybe thousands, of different proteins. A particular family, called neurexins, has attracted increasing attention because it appears to breed master regulators of synaptic organization and assembly in mammals.
Researchers have evidence that neurexins mediate trans-synaptic signaling and shape neural networks. At the same time, studies hint at an intriguing mechanism to achieve diversity and specificity in synaptogenesis. On the clinical front, investigators have linked mutations in genes encoding neurexins to autism, Alzheimer’s disease and schizophrenia, as well as depression, substance abuse, even obesity.
While this excites molecular neurobiologists, particularly those keen on the workings of synapses, no one knows precisely what specific neurexins are doing, partly due to a lack of the right protein tools.
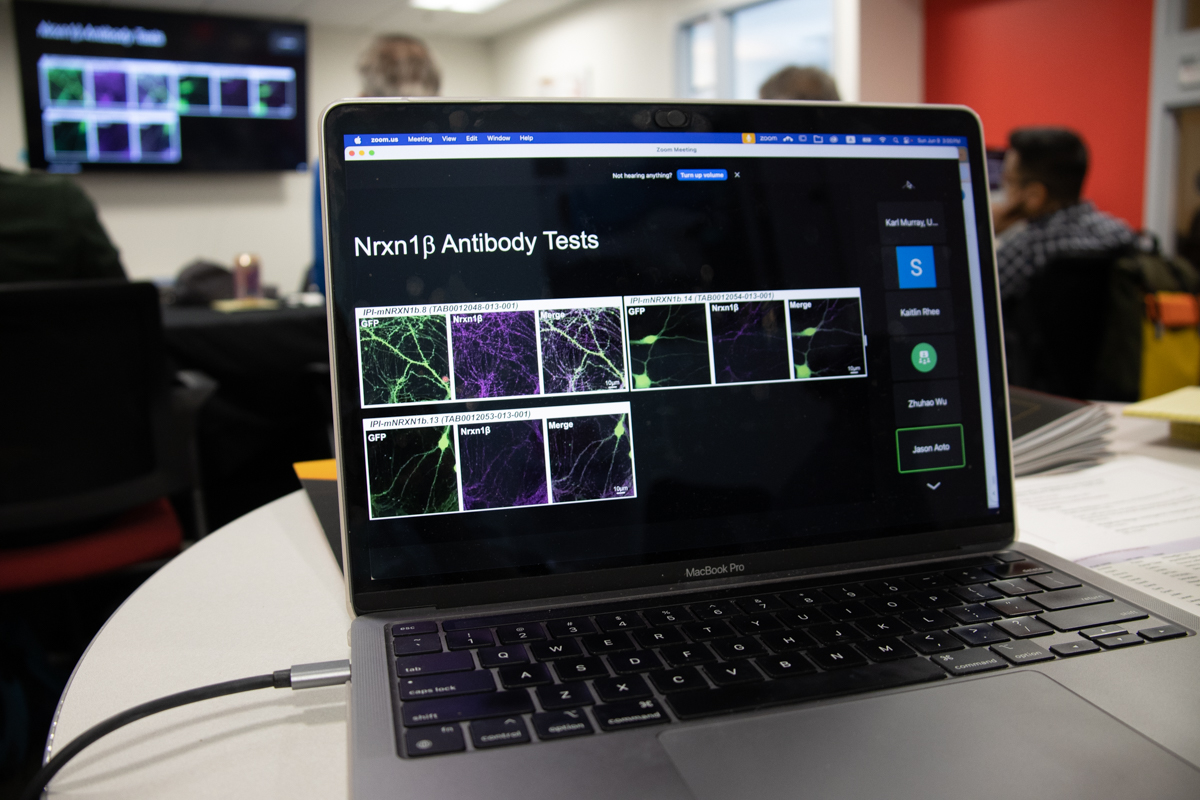
“One of the major limitations in the field of fluorescent neurology is a lack of specific antibodies,” says Jason Aoto, a neurexin researcher at the University of Colorado and IPI collaborator. “Many groups have tried to make antibodies for neurexins, but the best thing is an intracellular antibody that detects all neurexins.”
As part of its Neuroscience Program, and sponsored by a grant from the Chan Zuckerberg Initiative, the Institute for Protein Innovation (IPI) is developing antibodies for specific neurexin family members and distributing them to researchers, such as Aoto, to test their performance in specific neurobiology applications.
The results, thus far, look promising. At a workshop hosted by IPI in Chicago in October, Aoto showed the specificity and utility of IPI antibodies in 3D STORM imaging and demonstrated their promise compared to much more complicated V5 tagging approaches.
“It does seem like there is specific labeling,” he says. “But we need to validate further to be sure.”
How do neurexins work?
Neurexins are an enticing puzzle. Named for their discovery as a receptor binding a neurotoxin in the venom of the black widow spider, neurexins are essential evolutionarily conserved synaptic adhesion molecules.
The family comprises three genes in vertebrates — NRXN1, NRXN2 and NRXN3 — and homologs in invertebrates. The proteins exist in all model organisms: flies, worms, fish, frogs, among others, and even in sponges and jellyfish, although neurexins’ function is a mystery in organisms that lack synapses.
In mammals, each neurexin gene encodes an α-neurexin with a long extracellular portion and a β-neurexin with a shorter extracellular portion. These proteins extend across the cell membrane and interact with the extracellular matrix primarily from their base location on the neuronal surface.
Because neurexins protrude into the synaptic cleft, they have the unusual ability to form trans-synaptic bridges with different partners. This unique structure enables them to physically clump cells together and, at the same time, engage in intracellular signaling. This bidirectional signaling allows neurexins to control presynaptic neurotransmitter release and post-synaptic function.
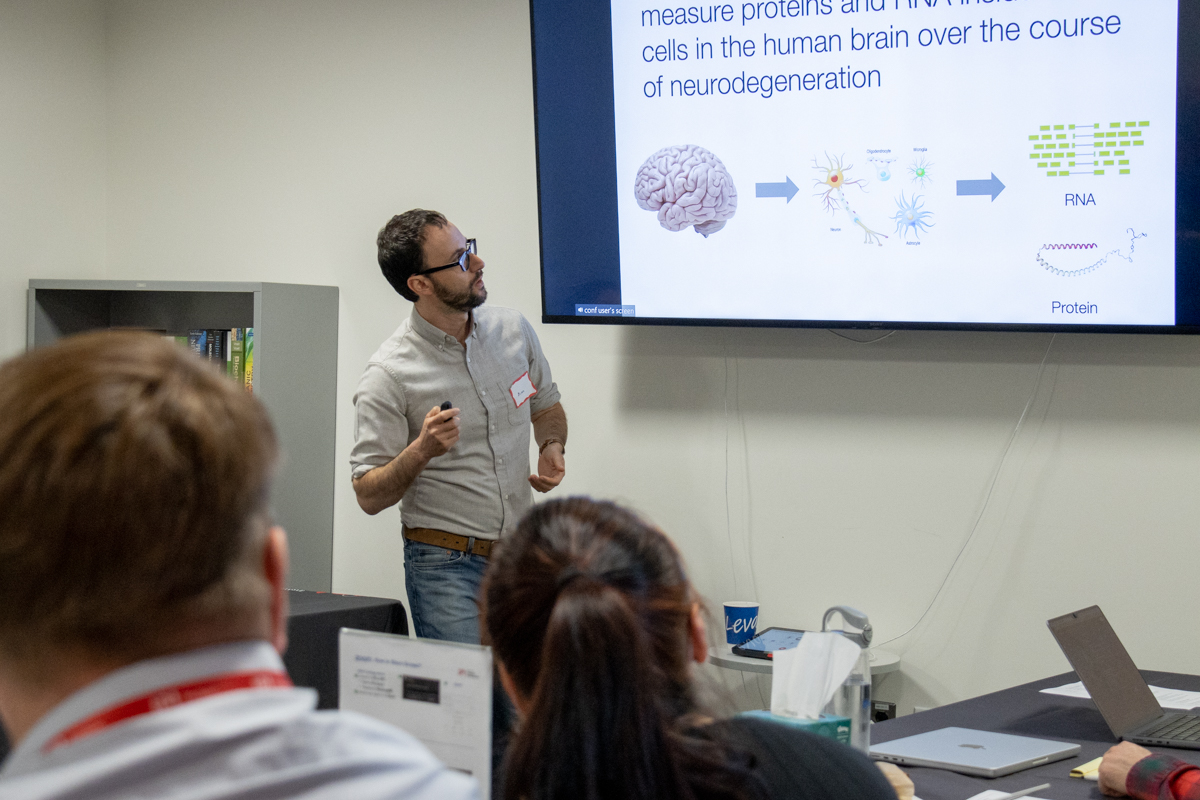
Adding more feats to their performance, neurexin’s three vertebrate genes include five conserved alternative-splice sites in the α-neurexin coding sequence and two in β-neurexin, allowing for almost 4,000 possible neurexin forms with distinct structural domains and flexibility. Neurexin isoforms are essential for Ca2+-dependent transmission for excitatory and inhibitory synapses, each with a different expression pattern and slightly varied function in the brain.
Stanford neuroscientist Thomas Sudhof, a pioneer in neurexin research and a 2013 Nobel Prize winner, notes that despite the importance of neurexins and the thousands of papers published on family members, they remain poorly understood. This gap is due mainly to neurexin’s many variants, vast numbers of interactions and divergent functions.
Adding to the complexity, specific neurons differentially express neurexins’ ligands in synapse-specific localization patterns. The combination of both ligand and neurexin diversity yields an enormous array of synapse properties and potential.
“There’s a huge molecular code, and nobody understands how it works,” says Rob Meijers, IPI senior director of the antibody platform.
That is why IPI is working on making antibodies targeting the six main members of the neurexin family and some variants, including an antibody to a particular splice variant: neurexin-1α, deletions of which have been linked with a significantly elevated risk for autism spectrum disorder.
Why are neurexins so tough to target with antibodies?
One main reason for the dearth of isoform-specific neurexin antibodies is their evolutionary conservation.
“If you inject a mouse with human neurexin, the mouse has a similar neurexin in its brain, so it’s not going to make antibodies against it,” Meijers says.
One workaround is genetically modifying the mouse so its neurexins carry a traceable peptide tag, such as Aoto’s V5-tagged neurexin example. But tagging leads to all kinds of problems. Added peptides can perturb protein folding, binding or function, for example. And researchers cannot use more than one tag without extreme complication. Researchers are considering cross-breeding mice with differently tagged neurexins just because there are no good antibodies for this protein family, Meijers says.
Researchers are forced to use genetic techniques, tweaking genes to switch neurexins’ expression on and off in select brain sections, a technique akin to using a sledgehammer when circumstances call for a scalpel.
What antibodies does IPI have?
Using synthetic biology, IPI has created antibodies to the entire neurexin family, including neurexin-1α/β, 2α/β and 3α/β, targeting epitopes that protrude into the extracellular part of the membrane. These antibodies are currently undergoing beta testing by collaborating researchers; qualified researchers interested in joining IPI’s community validation effort can contact IPI for more information.
What is the potential payoff?
The availability of isoform-specific antibodies should help researchers such as Dima Ter-Ovanesyan, a senior scientist at Boston’s Wyss Institute, currently collaborating with IPI. His team is studying ways to use biofluids from extracellular vesicles as a source of biomarkers to potentially diagnose neurodegenerative diseases such as Parkinson’s and Alzheimer’s.
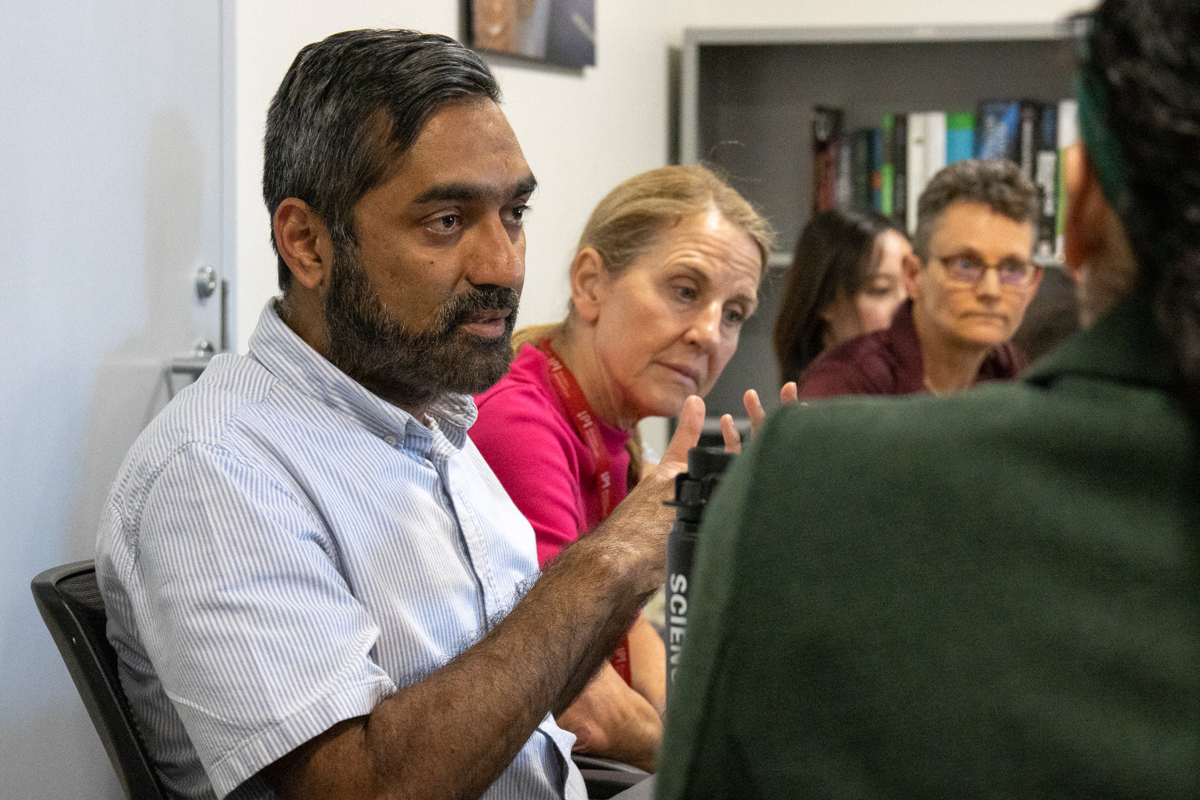
“The dream,” Ter-Ovanesyan says, “is to take a blood sample, pull out proteins that come from the brain, and then analyze them. It’s like a window into the brain.”
For this work, it’s crucial to have antibodies specific and sensitive enough to zero in neuron-specific extracellular vesicles containing neurexin-3. The best commercially available antibodies are polyclonal, which means they are more likely to cross-react with other molecules, vary between batches and eventually run out after the animal making the polyclonal dies.
Ter-Ovanesyan says his lab is “very excited” at the prospect of having access to antibodies that will be superior in affinity and specificity to anything currently available.
Meanwhile, Tabrez Siddiqui, an IPI collaborator at the Max Rady College of Medicine at the University of Manitoba, is interested in neurexin-3 in relation to obesity and addiction. Particularly, his team wants to unravel the synaptic mechanism of drug addiction.
“We are increasingly recognizing that different neurexin variants have shared functions,” he says, “but they also have unique functions. So it’s very important that we are able to address this diversity by having reagents that can target individual isoforms.”
Meijers notes that IPI is currently developing antibodies to components in the synapse — not only neurexins but also many other pre- and post-synaptic proteins. Digging into the molecular composition of synapses could help identify small molecules that can boost certain synapses as needed or try to influence how synapses are pruned or deleted, ultimately unlocking a way to reverse the symptoms of Alzheimer’s or autism.
While Siddiqui acknowledges that this scenario is still a dream, he says IPI is doing a “huge service to the community” in developing antibodies targeting synaptic proteins and reaching out to the neurobiologists for validation.
“Who knows,” he says, “some of these antibodies could become therapeutics.”
IPI is currently recruiting beta testers for our neurexin and neuroligin antibodies, as well as other neuroscience-related families, including netrin, DCC, Robo, Slit and semaphorin. Contact us at antibodies@proteininnovation.org for more information on our validation program.
Sources:
Jason Aoto, jason.aoto@cuanschutz.edu
Rob Meijers, rob.meijers@proteininnovation.org
Tabrez Siddiqui, tabrez.siddiqui@umanitoba.ca
Dima Ter-Ovanesyan, dter@wyss.harvard.edu
Writer: Deborah Halber, dhalber@writingreality.com
About IPI
The Institute for Protein Innovation is pioneering a new approach to scientific discovery and collaboration. As a nonprofit research institute, we provide the biomedical research community with synthetic antibodies and deep protein expertise, empowering scientists to explore fundamental biological processes and pinpoint new targets for therapeutic development. Our mission is to advance protein science to accelerate research and improve human health. For more information, visit proteininnovation.org or follow us on social media, @ipiproteins.