Hundreds of millions of years before humans evolved, a family of proteins came to be — first in worms, next in flies, then in mammals. The proteins, called glypicans (or GPCs, for short), evolved to perform a task: directing the signals passed by growth factors during development.
Glypicans were good at their jobs, and cells resourcefully slotted GPCs into myriad systems: nudging neuron migration in worms, patterning the wings of fruit flies, fostering synapse formation in rats and many more.
Indeed, glypicans are everywhere, in nearly every tissue in the human body and all other animals.
“This is a very unique family that can interact with a huge and wide repertoire of ligands,” says Daniel del Toro at the University of Barcelona in Spain. “They regulate so many processes at many different time points. We have only seen the tip of the iceberg.”
Now, 35 years after the discovery of glypicans, the Institute for Protein Innovation (IPI) has created a suite of antibodies targeting members of the human glypican family to better interrogate their many functions. The antibodies can help researchers probe the workings of glypicans in early development, brain wiring and synapse formation — with implications for cancer, developmental syndromes and neurological diseases.
How do glypicans work?
To grasp glypicans’ function, it’s necessary to understand their structure. Glypicans are part protein, part sugar and part lipid. The lipid, called glycosylphosphatidylinositol (GPI), anchors the glypican to the cell surface; it is a loose attachment, enabling glypicans to skate over the cell surface and hunker down in “microdomains,” self-organized dynamic islands of the plasma membrane.
This positioning puts glypicans dead center in gatherings of various key receptors on the cell surface. There, glypicans harness their structure — long sugar polymers, immutably fixed to a protein core. The sugars, called glycosaminoglycans (GAGs), are sticky, due to their many negative charges.
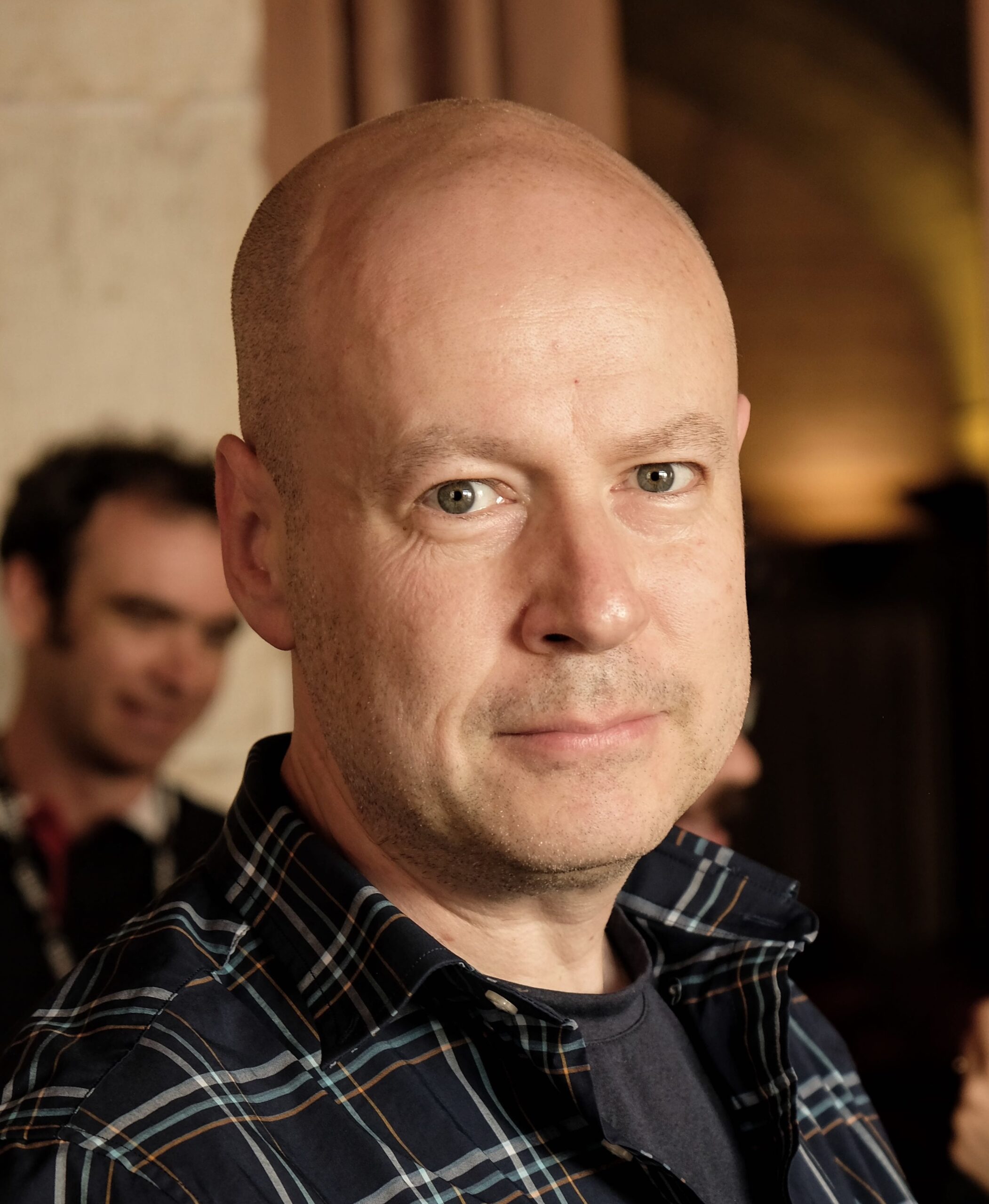
“Glypicans soak up signaling proteins from outside the cell,” says Adrian Salic at Harvard Medical School. In this manner, glypicans concentrate signaling proteins on the cell surface, helping them trigger their effects on the target cell.
What does all this mean for cells?
The first clues came from fruit flies. In 1995, Drosophila researchers identified a protein called Dally resembling mammalian glypicans. Flies lacking Dally failed to develop wings, linking Dally to what was named the wingless pathway, which is triggered by a signaling protein called Wg.
While notably lacking in wings, humans do have a Wg counterpart called Wnt-1, a glycoprotein pivotal in an ancient pathway that controls embryonic development. It seemed that glypicans might be acting in concert with conserved receptors regulating development across species.
In that vein, five years after Dally’s discovery, Drosophila researchers uncovered a Dally-like protein (Dlp), also crucial in development. Even more glypican family members, called paralogs, turned up. Researchers found that, moving up the evolutionary chain, worms have one glypican, flies have two and mammals carry six.
The increasing number matches the complexity of the organism — but comes with some redundancy. GPC3 and 5 most resemble each other (and Dally); GPC1, 2, 4 and 6 seem closer to Dlp. Though their functions overlap, glypicans work together to fine tune up or down signals that control communication between cells.
How related are glypican family members?
Given this family tree, it may seem odd that glypican members today don’t look alike, both between animal species and between paralogs of the same species. During hundreds of million years of evolution, the sequence of glypicans has diverged considerably. For example, Dally and GPC1 share as little as 20% sequence identity.
However, glypican crystal structures clearly show their relatedness. All glypicans carry 14 cysteine residues localized in nearly identical locations. The proteins contort into 14 alpha helices and bend into three primary loops with the GPI anchor at the protein’s tail, embedding the receptor into the plasma membrane.
This three-dimensional structuring allows glypicans to fit in — literally. They ease into the pockets and interfaces of several morphogens and growth factors, including bone morphogenic proteins (BMPs), hedgehog proteins (Hhs) and fibroblast growth factors (FGFs). These diverse interactions with highly conserved developmental pathways explain the dizzying complexity of glypican roles.
Some tissues express one glypican family member. Other tissues another. Some glypicans boost signaling. Others damp it down. The same glypican in the same pathway can promote or prevent signal transmission depending on context, such as its expression level, tissue type, timing or the concentration of growth factor.
“The power of these pathways is that they are very versatile,” says Salic. It’s evolution 101: “The pathway broadens its scope simply by making more paralogs of each component and playing this spatial-temporal expression game.”
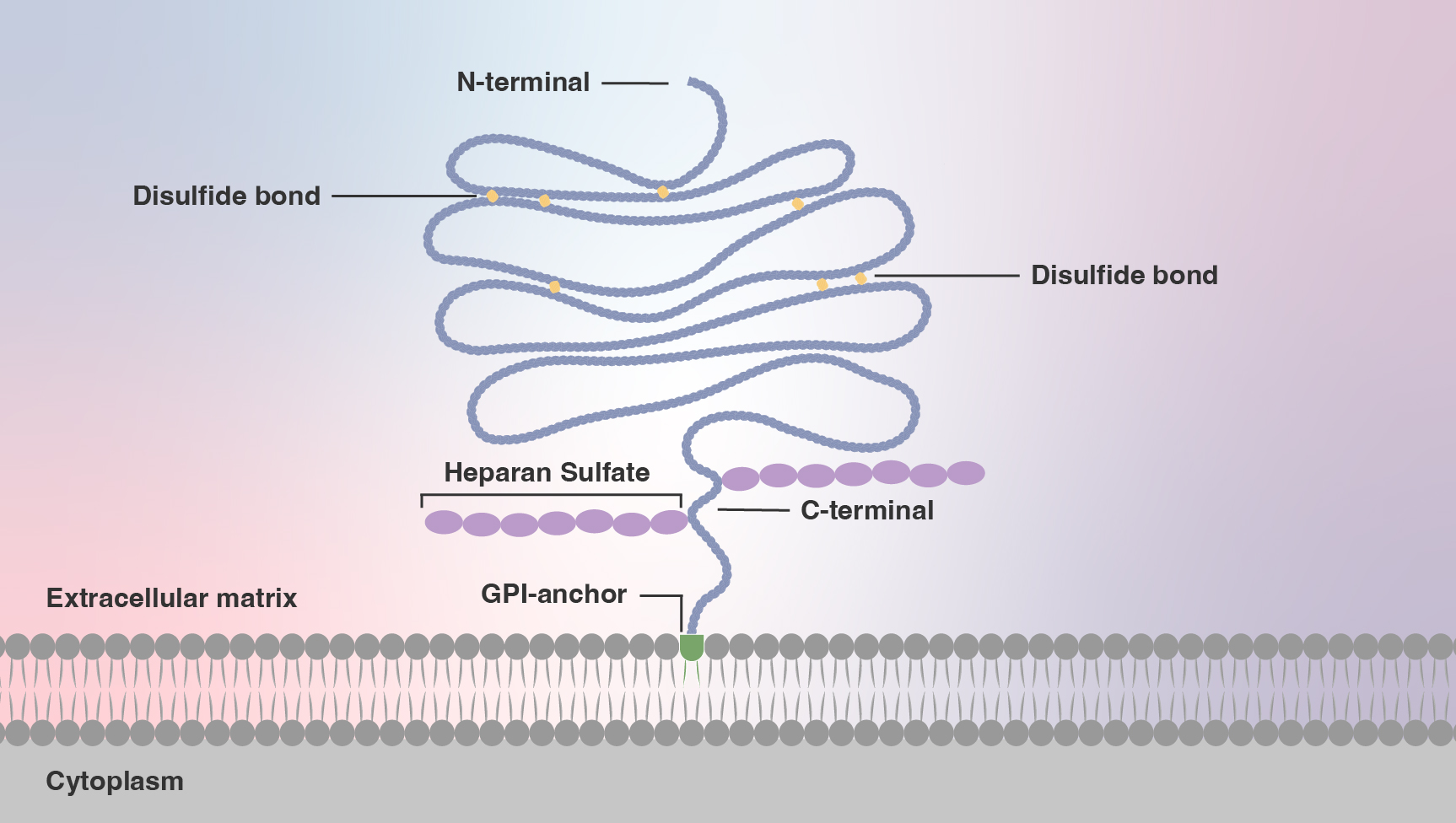
How are GPCs featuring in research and therapeutics?
That game is now captivating researchers. Glypicans “are very, very interesting, and there is so much that is not known,” says del Toro. Beyond development, glypicans are emerging as significant players in neurobiology.
For instance, fly researchers showed that Dlp guides the interaction of a receptor-ligand pair known as Robo-Slit during axon guidance, the process by which neurons send out axons to reach their correct targets. GPC4 acts as a glue within synapse-organizing protein complexes, helping maintain excitatory synapse development and function. In the journal Cell, del Toro and collaborators showed that GPC3 forms complexes with a receptor called uncoordinated receptor 5D (Unc5D), to guide migrating pyramidal neurons in the mouse brain.
Meanwhile, glypicans can also act in cancer.
Del Toro’s colleagues demonstrated that the same Unc5/GPC3 complex coaxing neuronal migration during embryogenesis also steers cancer cells in a mouse model of neuroblastoma. Glypicans are also “re-expressed” in pediatric solid tumors and in pancreatic cancer. Other labs have shown that, in contrast to normal adult liver cells, approximately 75% of liver cancer cells express GPC3. This occurrence has led researchers to explore GPC3 as a diagnostic and therapeutic target for hepatocellular carcinoma, the most common type of primary liver cancer.
How can antibodies help?
These new avenues make glypicans an exciting area of research, and antibodies can be key tools. By specifically targeting glypican family members, antibodies help elucidate novel mechanisms and drug targets.
“Glypicans are important in so many different fields from development to cancer biology to synapse formation,” says del Toro. “With the tools being made and the new knowledge we are gaining, (researchers) have opened doors to do experiments that before were not possible.”
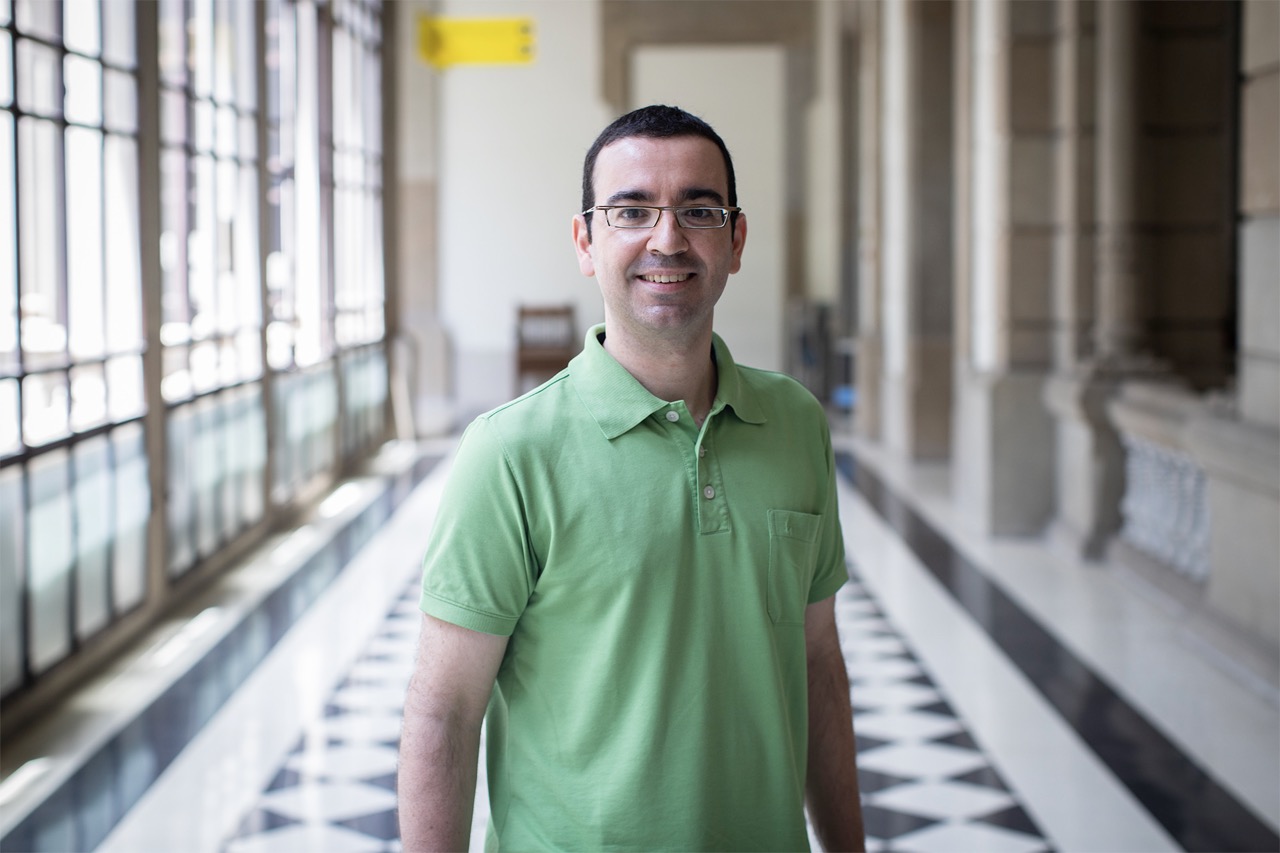
IPI is now providing some of those necessary tools, including antibodies to GPC1, 3 and 4.
The IPI glypican antibodies were discovered using a yeast display platform that allowed for fine-tuned sorting against antigens developed in-house. The resulting panels of antibodies went through extensive biophysical characterization at IPI, as well as additional external evaluation and application testing performed by collaborating glypican researchers. Following large-scale production, the IPI team used cell surface display and immunofluorescence to determine species reactivity and pinpoint GPC specificity.
By making these antibodies available through Addgene, IPI director of commercialization Mike Walden says the Institute aims to propel glypican research beyond its current frontiers, while emboldening a community approach to antibody production.
“This release continues our commitment to ensure IPI antibodies are highly characterized both by IPI researchers and experts from around the country,” Walden says, noting that researchers can find this data and antibody sequences through the Addgene Data Hub. “We strive to provide the highest degree of transparency that can be found in the market.”
Sources:
Mike Walden, mike.walden@proteininnovation.org
Adrian Salic, adrian_salic@hms.harvard.edu
Daniel del Toro, danieldeltoro@ub.edu
Writer: Trisha Gura, trisha.gura@proteininnovation.org
About IPI
The Institute for Protein Innovation is pioneering a new approach to scientific discovery and collaboration. As a nonprofit research institute, we provide the biomedical research community with synthetic antibodies and deep protein expertise, empowering scientists to explore fundamental biological processes and pinpoint new targets for therapeutic development. Our mission is to advance protein science to accelerate research and improve human health. For more information, visit proteininnovation.org or follow us on social media, @ipiproteins.